BARDEEN RESEARCH GROUP
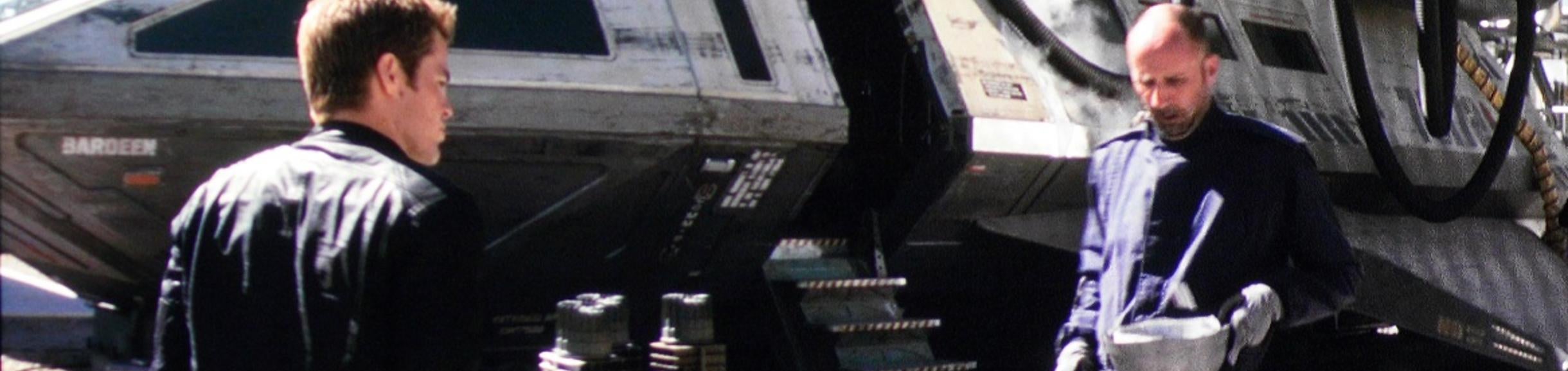
Research
Photomechanical Material Systems—From Molecules to Devices
Office of Naval Research MURI Award
Team (in alphabetical order):
Chris Bardeen @ UC Riverside
Kaushik Bhattacharya @ Cal Tech
Ryan Haywood @ University of Massachusetts Amerherst (PI)
Peter Palffy-Muhoray @ Kent State University
Todd Martinez @ Stanford University
Javier Read de Alaniz @ UC Santa Barbara
Materials that convert light into motion, mechanical work, and changes in shape have long captured the imagination of scientists and engineers for their potential in devices that are powered by light and controlled by optical signals. However, even the most advanced materials developed to date lag far behind systems that operate on traditional chemical or electrical power sources. This project aims to develop the fundamental understanding needed to design molecules and material architectures that efficiently convert photon energy into mechanical work.
Understanding How Reaction Kinetics and Morphology Affect Photomechanical Molecular Crystals
Division of Materials Research NSF
Principal Investigator
The creation of stable photoreactive molecules is a prerequisite for better performing materials. One phase of the research concentrates on making new molecules to make crystalline structures that can survive exposure to air and solvents. For example, the use of fluorine substitution will raise the molecular oxidation potential and may also enhance crystal plasticity. Molecular crystals can also exhibit nonlinear spatio-temporal reaction kinetics that can lead to autocatalysis, enhanced mechanical response, and oscillatory motion under steady-state illumination. Oscillatory motion can potentially be harnessed to provide locomotion for micro-swimmers. Along with new theoretical approaches, a novel standing-wave fluorescence experiment will be developed to directly probe the nonlinear reaction kinetics in single crystals. Finally, both bottom-up solution growth and top-down laser cutting will be used to create crystals with well-defined orientations and shapes. By controlling both crystal shape and the orientation of the strain tensor with respect to that shape, a detailed investigation into how both variables determine the crystal's response to light will become possible.
2D Encapsulation of Molecular Crystals for Close-contact Measurement of Exciton Dynamics
Division of Chemistry NSF
Principal Investigator
The project focuses on prototypical molecular crystal semiconductors like perylene, tetracene ,and diphenylhexatriene. Plate-like molecular crystals with variable thicknesses are grown and then covered with graphene and hexagonal boron nitride. With this enabling technology in place, two categories of experiments are pursued. The first involves measuring the properties of the encapsulated crystal and its interaction with the environment using microscopy and photoluminescence methods. Questions that can be addressed include whether the exciton behavior in molecular crystals heterogeneous on length-scales down to 20 nm and whether it is possible to transfer energy and charge across the atomically thin membranes. The second category of experiments focuses on new space and time-resolved experiments to determine whether spin-entangled states of triplet pairs produced by singlet fission can extend over mesoscopic distances.
RAISE-TAQS: Nonlinear Optical Properties and Novel Quantum Phases of Polar Molecules in Optical Lattice
Division of Physics NSF
Co-PI w/ Boerge Hemmerling (PI) & Shan-Wen Tsai (Co-PI)
The main objective of this project is to load a quantum degenerate gas of polar AlCl molecules into an optical lattice to study novel quantum phases and simulate solid-state crystalline materials. This research has four specific goals: First, perform precision measurements of molecular properties, such as the energy spectrum and the Franck-Condon factors of AlCl; Second, cool the polar AlCl molecules to quantum degeneracy and study the dynamics and stability of dipolar Bose-Einstein condensates. The group will also explore correlated phases due to the anisotropic dipole-dipole interaction, including liquid crystal and density-wave phases, and probe the interplay between superfluidity and charge-ordering in low-dimensional materials; Third, create a one-dimensional optical lattice of polar molecules and study its non-linear optical response by measuring the second harmonic generation. By varying temperature and ordering, the optical lattice can be tuned from the limit of independent, noninteracting molecules to a strongly correlated one-dimensional crystal; Fourth, use Raman scattering to explore the transition from intramolecular vibrations of noninteracting molecules to intermolecular phonon structure by continuously tuning the lattice parameters. The experiments will demonstrate that ordered structures of diatomic molecules can exhibit phenomena that are directly relevant to solid-state materials. These results will motivate future experiments to expand the lattice to two dimensions and finally to fully mimic three-dimensional crystal systems. Their low temperature and tunable structure could lead to totally new phenomena, like giant nonlinear optical susceptibilities, that could inspire new research directions in the field of "crystal engineering".